Physics studies at the Large Hadron Collider
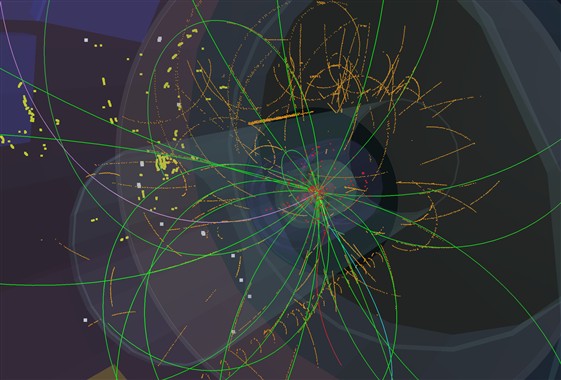
Particle physicists are hoping to have answers to a number of questions from the experiments at the Large Hadron Collider (LHC). Some of these questions are discussed below.
What makes things heavy?
Any theory of particle interactions must combine the physics of the very small (quantum mechanics) and the physics of the very fast (special relativity). In the simplest theory that satisfies the various constraints, all particles have zero mass. Experimentally, this isn’t true. The theory can be improved by introducing a new type of field, analogous to an electric or magnetic field, but present everywhere. This is the Higgs field, named after Peter Higgs, who played an important part in the theoretical developments. The mass of a particle is then proportional to the resistance felt in moving through the Higgs field. The field has an associated particle, known as the Higgs boson, that hasn’t yet been detected. Discovering the Higgs boson is one of the major objectives of the LHC experiments.
Why are there no anti-worlds?
All known processes for particle creation produce matter and antimatter in exactly equal amounts. This suggests that the Universe itself would have been created as equal amounts of matter and antimatter, which might have been expected to annihilate one another, leaving only energy in the form of heat and light. This clearly didn’t happen, and the visible Universe today consists almost entirely of matter. Earlier experiments have measured subtle differences in the way that matter and antimatter behave. Differences of this type might explain how the Universe has evolved to its current state, but the observed differences are too small to account for the near-total absence of antimatter. Experiments at the LHC are looking for further matter-antimatter differences, to help solve the puzzle.
What flavour is quark-gluon soup?
Head-on collisions between lead ions at the LHC can produce, in a tiny volume, matter and energy densities similar to those thought to have existed a few millionths of a second after the Universe was created. Under these extreme conditions, the lead nucleons dissociate into their component parts. The unbound quarks and gluons then form a primordial state of matter known as quark-gluon plasma or, more informally, as quark-gluon soup. This state of matter, and its transition to hadrons, is being studied by the LHC experiments to learn more about the workings of the strong force. Primary aims include understanding how quarks become confined in hadrons, and understanding if the confinement mechanism generates contributions to hadron masses.
What keeps galaxies spinning?
Stars in a galaxy move in orbits about the galactic centre. Based on the distribution of visible matter, stars nearer the centre would be expected to move faster than stars further out. Measurements over many galaxies suggest that this isn’t the case, and orbital speeds are almost independent of star-to-centre distance. The most widely accepted interpretation is that the gravitational effect of the visible matter is supplemented by the effect of additional matter, given the name dark matter, which doesn’t emit or reflect light. This dark matter is estimated to account for about 83% of the total mass of the Universe. There are hints from astronomical observations that dark matter is composed of unknown particles that, like neutrinos, interact only through the weak force. These particles could be found by the LHC experiments.
Where's SUSY?
Supersymmetry, often abbreviated as SUSY, is a theory that relates fermions and bosons. It requires the existence of currently undetected particles, known variously as SUSY particles, superpartners, and sparticles. Each of the fundamental fermions (quarks and leptons) should have a boson superpartner, and each of the fundamental bosons (force carriers) should have a fermion superpartner. Supersymmetry has been widely studied as part of possible extensions to the Standard Model of Particle Physics, and has some appealing features. For example, it allows the weak, electromagnetic and strong forces to be understood as having a common origin. The LHC experiments are searching for evidence of SUSY particles, which may have high masses. The lightest SUSY particle could be a candidate for dark matter.
Why do apples fall so slowly?
Isaac Newton recounted how his idea of gravity “was occasion’d by the fall of an apple”. A puzzle that remains is why apples fall so slowly, or, equivalently, why gravity is so feeble compared with other forces. Some theories suggest that gravity’s apparent weakness comes from its strength being spread across more dimensions than the three space dimensions and one time dimension experienced in everyday life. In collisions at the LHC, energy could potentially be carried to the extra dimensions by gravitons. The effect of extra dimensions may then be visible to the experiments as interactions with large energy deficits.
Can black holes be made at home?
A black hole is a region in space where the escape of anything that enters, including light, is prevented by the region’s gravitational field. Astronomers have found black-hole candidates that could have formed from collapsed stars, but microscopic black holes are theoretically possible also. With three spatial dimensions, the energy needed to create a microscopic black hole is far beyond the reach of any particle accelerator that could be built with today’s technology. However, the existence of extra dimensions would be likely to reduce the energy requirement, so that microscopic black holes might be produced and detected in LHC interactions. Any such black holes would be expected to evaporate almost instantly, radiating particles and antiparticles through a mechanism first proposed by Stephen Hawking. If microscopic black holes are produced at the LHC, then they’re also regularly produced around the Earth in cosmic-ray interactions.
What does nobody know?
Although the LHC experiments have been designed to help answer many intriguing questions, one of the most exciting prospects of all is that they will discover something completely unexpected.